As talented people were drawn to the cities and into business, agricultural markets expanded, pressuring agricultural productivity. Town records show common-field smallholders actively experimenting with plant varieties and crop rotation schemes to improve output. A British empirical, scientific style of thinking became a norm. And wages rose. By 1800, British wages, measured by both exchange rates and purchasing power, were the highest in Europe by a wide margin. Processes that moved at a glacial creep in the sixteenth century, coalesced and accreted in the seventeenth, and finally exploded in the eighteenth.
j
6
Cotton textile manufacture was the quintessential industry of the British Industrial Revolution. From a small cottage enterprise in the early
1700s, it had grown by 1830 to employ 425,000 workers, accounting for one out of every six manufacturing jobs and about 8 percent of GDP. But the burst of mechanical development that created the world's first mass-production factories was preceded by seventy-five years of diligent tinkering.
7
Yarn spinning is a quintessentially hand-labor task. Cotton fibers are delicate. To use in cloth, they first must be cleaned and straightened; then a “spinster,” using the traditional spinning wheel, would repeatedly draw the fibers out under finger pressure while twisting them for strength. Drawing up machinery to replicate hand-spinning was relatively straightforward, but actually building machines that could manipulate the threads more or less as humans did, and without breaking them, took years of trial-and-error experiment. The first successful spinning machine was the jenny, built in 1767 and driven by a hand crank. James Hargreaves, a weaver, worked out a method of holding the rough cotton, or roving, with pins, while a bar stretched out and twisted the fibers. It worked well enough that an early demonstration provoked a riot by the local hand-spinners. But by the 1780s, many cottage hand-spinners were using twelve-and twenty-four-spindle jennies. A twelve-spindle jenny cost about seventy times as much as a spinning wheel, so the productivity pickup must have been very high.
Richard Arkwright was right behind with the water frame, a spinning machine designed to be water-powered. Arkwright was more entrepreneur than inventor, and he was determined to create a cotton mill industry. He bought patents and hired craftsmen to come up with the machinery. His water frame applied rolling technology similar to that used in glass and metal working. The rovings were fed into three successive rollers: the first compressed them, while the second two, each running at progressively higher speeds, both compressed and stretched the fibers as another device twisted them. Arkwright was far from the first inventor to try rollers: the challenge was getting the speeds, the pressures, and the spacing between the rollers right. Arkwright also had the insight to hire clockmakers to build his machines, for they had the most contemporary experience with precision gearing.
Â
The foot-treadle, flying shuttle, heddle loom achieved very high manual production rates. At each push of the treadle, the heddles raised and lowered alternate warp (long) threads, creating a shed to pass the weft (cross) thread through, and the batten pushed the new weft thread firmly into place. The flying shuttle allowed the operator to remain seated and very rapidly pull the weft threads back and forth through the alternating sheds. Mechanized looms worked exactly the same way, but at much higher rates of speed.
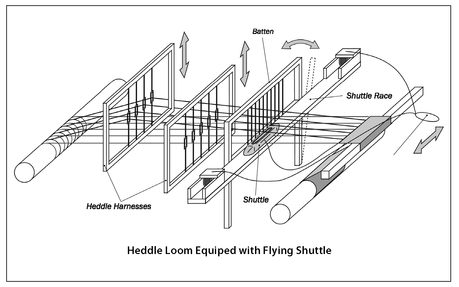
By roughly the same methods, Arkwright produced the first successful “carding” machineâfor combing out the raw cottonâand then spent most of a decade trying to build a cotton mill. Cotton mill management was a new discipline: it required learning how to run banks of the new machines efficiently, how to lay out the work flow, and how to manage machined cotton. The stages from machined rovings to finished yarns were subtly different from those of hand-spun cotton. Knowing what machine speeds to apply with different fibers and spotting when a yarn was about to break, knowing how to intervene and how to restart equipment after a disasterâin effect, the basic textbook of mill managementâhad to be invented from scratch. Arkwright's second major mill got most of it right and was the prototype for a new British industry.
Mechanization in the textile industry was an alternating race between spinning and weaving. The fly-shuttle foot-pedal heddle loom was a highly
rationalized machine that quickly pressured the capacity of the hand-spinning industry, forcing the pace of mechanization. Mechanized spinning shifted the pressure back to weaving. The fly-shuttle loom almost cried out for mechanization; the challenge lay in tuning the pressures on the threads to produce acceptable cloth while minimizing breakage, the way a skilled human did by feel.
It was much the same with the steam engine. Galileo's pupil Evangelista Torricelli did much of the early basic science, and a Frenchman, Denis Papin, constructed early working models. The first useful industrial-scale steam engine was built by Thomas Newcomen in 1712 to lift water out of a tin mineâflooding of underground mines was a chronic problem. It used a vacuum to produce work. Steam entered a cylinder and raised a piston; a jet of water cooled the cylinder, and the steam condensed, causing the piston to fall, and thereby lift water.
Very little is known about Newcomen, except that it took him at least ten years to work out his concepts, and he was forced to share his patent with businessmen who bought a master patent on all “fire-engines” from the estate of Thomas Savery, who had failed to develop a working engine. But Newcomen's invention is a marvel. Instead of trying to lift water directly by the piston, he used it to drive an oscillating pump handleâthe walking beam engine. All prior engine models required an attendant to change valve settings at each stroke, but Newcomen operated his valves from the beam motions, possibly the first self-acting engine. His method of piston sealing was standard for many years. Newcomen-type engines spread rapidly through the British and European mining industry, although their inventor may have earned little from them.
Newcomen-type engines reigned for a half century, until James Watt engineered another design leap. Watt was an instrument maker who was asked to build a model engine for teaching purposes at the University of Edinburgh. As he worked on it, it occurred to him that the engine's use of fuel was prodigious relative to its output, and he calculated that three-quarters of the fuel was spent reheating the cylinder after it had been cooled to create a vacuum. That was not a problem at coal mines, where fuel was almost a free good, but it limited the engine's potential usefulness elsewhere. Watt's solution was to create a separate condenser with an air pump: when the steam entered the cylinder, the air pump created a vacuum in the condenser; the steam then rushed to the condenser, and the piston fell, with only minimal change in the piston-cylinder temperature. The resulting engine was more complicated but far more efficient, and it would operate at low steam pressures, simplifying boiler construction.
Â
The Newcomen engine used atmospheric pressure to do work. The right side of the walking beam (large wooden horizontal piece at top) acted as a pump handle that raised water as it was pushed down. In the starting position for a new stroke, the “pump handle” was tilted up by the weight of the pump rod on left. (The beam in the picture is shown in mid-stroke.) The work sequence was: 1) Steam fills piston cylinder. 2) Jet of cold water sprays outside of cylinder, condensing the steam and creating a vacuum. 3) The weight of the atmosphere pushes pump handle down and lifts water. 4) Weight of the pump rod returns handle to initial position. Stroke rates were about twelve per minute; boiler technology limited pressures to only one or two pounds per square inch.
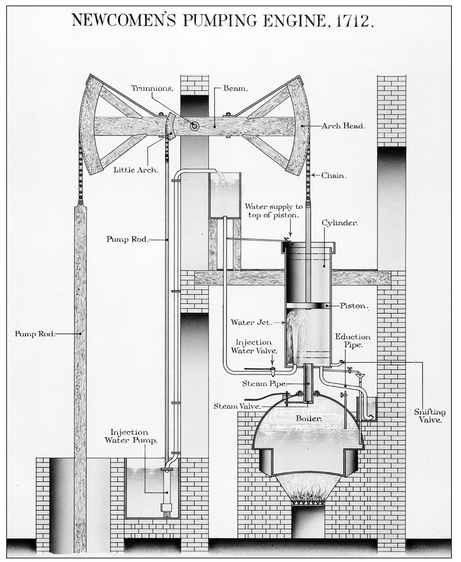
Watt did little with his engine until he partnered with Matthew Boulton in 1774. Boulton was a small metal product manufacturer and an honest man who convinced Watts that he had a global business opportunity. With Boulton in charge of the business side, and Watt steadily improving his designs, the partnership sold hundreds of engines throughout Great Britain and abroad. Boulton and Watt both retired when their patents expired in 1800, turning over the business to their capable sons. Over the rest of the nineteenth century, Boulton & Wattâtype engines were gradually supplanted by lighter and less expensive high-pressure engines, developed independently by Richard Trevithick in Great Britain and Oliver Evans in the United States. Boulton & Wattâstyle engines kept a foothold well into the nineteenth century, wherever safety and fuel efficiency were dominant concerns, as in transatlantic steam ships.
8
By much the same processes, the British also came to dominate in iron and steel, although the critical innovations were powered by dire necessity. Iron and steel were among the most ancient of products, and in the first part of the eighteenth century, the Germans, Swiss, and French all vied for leadership. The British pulled ahead by the century's end, even though they started from a huge disadvantage. Iron processing requires vast amounts of fuel, traditionally charcoal, almost always used in direct contact with the ore or metal. By the eighteenth century, however, the British industry was close to exhausting its wood supplies and was forced to fall back on coal, which is high in impurities that can be fatal to iron quality. The process of overcoming that obstacle made the British industry by far the most technically sophisticated in the world. Key developments
were the use of coke, a purified form of coal,
k
as a charcoal substitute; the development of the reverberatory furnace, which melted iron without direct contact with the fuel; and the puddling process, a deeply skilled craft using long poles to stir melted iron from a reverberatory furnace to create quality wrought iron. Very little of this qualified as new invention; it was instead accomplished by focused and sustained development of older technologies.
British iron prowess allowed the great machinist John Wilkinson to cast solid naval cannons and bore their holesâa formidable challenge. Other countries cast their cannon in molds, but they tended to explode. (Recall Isaac Chauncey's cannon debacle during the Burlington Races on Ontario.) A French expert reported to his government in 1775 that it had been twenty years since a British naval cannon had exploded, while French sailors “fear the guns they are serving more than those of the enemy.”
9
The crown jewel of British metallurgy was “crucible steel,” which was brought to a high state of perfection by Benjamin Huntsman in the 1740s near Sheffield, traditionally the British center for high-end steelmaking. Huntsman was trying to make very durable, very hard, very thin steel for clock and watch springs. The method he finally hit on entailed remelting quality conventional steel in clay “crucibles” or pots. The resulting product was a steel that was easily poured and cast and took a superb edge. Foreign competitors found the best Sheffield steel almost impossible to replicate. (The secret of the process, deduced only much later, was the local clay used in the pots.) A Swiss reported in 1778 that “the cast steel of England is, without contradiction, the most beautiful steel in commerce; it is the hardest, the most compact, and the most homogeneous; one can recognize it at a glance.”
10
The advances fed off each other. Lighter, more efficient steam engines were the perfect power source for textile factories, blast-furnace blowers, and the lathes, forges, trip-hammers, and other essential apparatus of big-ticket manufacturing. Bigger factories meant bigger machines running at higher speeds and tighter tolerances. High-speed engines impelled deeper inquiries into the physics and chemistries of metals and fuels, which fed into the knowledge base for soap production, bleaches, etching acids, and gas lighting for 24/7 factories. Sometime in the late eighteenth century, the British achieved a point of critical mass at which understanding expanded exponentially, and inventions, technologies, and accumulating lore cohered into an irresistible surge.