With rabbits and some other rodents, the necessary bacteria live in the large intestine. As the small intestine is where most nutrients are absorbed and the large intestine comes after the small intestine, these animals obtain the products from the cleaving of the β link by eating their feces. As the nutrients pass through the alimentary canal a second time, the small intestine is now able to absorb the glucose units released from cellulose during the first passage. This may seem, to us, a thoroughly distasteful method of coping with the problem of the orientation of an OH group, but it obviously works well for these rodents. Some insects, including termites, carpenter ants, and other wood-eating pests, harbor microorganisms that allow them to access cellulose for food, with sometimes disastrous results for human homes and buildings. Even though we cannot metabolize cellulose, it is still very important in our diet. Plant fiber, consisting of cellulose and other indigestible material, helps move waste products along the digestive tract.
STORAGE POLYSACCHARIDES
Though we lack the enzyme that breaks down a β linkage, we do have a digestive enzyme that splits an α linkage. The α configuration is found in the storage polysaccharides, starch and glycogen. One of our major dietary sources of glucose, starch is found in roots, tubers, and seeds of many plants. It consists of two slightly different polysaccharide molecules, both polymers of α-glucose units. Twenty to 30 percent of starch is made up of amylose, an unbranched chain of several thousand glucose units joined between carbon number 4 on one glucose and carbon number 1 on the next glucose. The only difference between amylose and cellulose is that in amylose the linkages are α and in cellulose they are β. But the roles played by cellulose and amylose polysaccharides are vastly different.
Part of the amylose chain formed from loss of H
2
O molecules between
α
-glucose units. The linkages are
α
as the -O is below the ring for C#1.
Amylopectin forms the remaining 70 to 80 percent of starch. It also consists of long chains of α-glucose units joined between carbons number 1 and number 4, but amylopectin is a branched molecule with cross-linkages, between the carbon number 1 of one glucose unit and carbon number 6 of another glucose unit, occurring every twenty to twenty-five glucose units. The presence of up to a million glucose units in interconnecting chains makes amylopectin one of the largest molecules found in nature.
Part of the structure of amylopectin. The arrow shows the C#1 to C#6
α
-cross-linkage responsible for the branching of amylopectin.
In starches, the α-linkage is responsible for other important properties besides our ability to digest them. Chains of amylose and amylopectin form into the shape of a helix rather than the tightly packed linear structure of cellulose. When water molecules have enough energy, they are able to penetrate into the more open helical coils; thus starch is water soluble whereas cellulose is not. As all cooks know, the water solubility of starch is strongly temperature dependent. If a suspension of starch and water is heated, granules of starch absorb more and more water until, at a certain temperature, the starch molecules are forced apart, resulting in a mesh of long molecules interspersed in the liquid. This is known as a gel. The cloudy suspension then becomes clear, and the mixture starts to thicken. Thus cooks use starch sources such as flour, tapioca, and cornstarch to thicken sauces.
The storage polysaccharide in animals is glycogen, formed mainly in the cells of the liver and skeletal muscle. Glycogen is a very similar molecule to amylopectin, but where amylopectin has carbon number 1 to carbon number 6 α-cross-linkages only every twenty or twenty-five glucose units, glycogen has these α-cross-links every ten glucose units. The resulting molecule is highly branched. This has a very important consequence for animals. An unbranched chain has only two ends, but a highly branched chain, with the same overall number of glucose units, has a large number of ends. When energy is needed quickly, many glucose units can be removed simultaneously from these many ends. Plants, unlike animals, do not need sudden bursts of energy to escape from predators or chase a prey, so fuel storage as the lesser branched amylopectin and unbranched amylose is sufficient for a plant's lower metabolic rate. This small chemical difference, relating only to the number and not to the type of cross-link, is the basis for one of the fundamental differences between plants and animals.
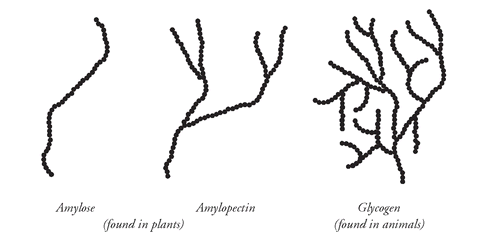
The different branching in starch (amylose and amylopectin) compared with glycogen. The greater the branching, the greater the number of chain ends for enzymes to break down the linkages and the faster glucose can be metabolized.
CELLULOSE MAKES A BIG BANG
Although there is a very large amount of storage polysaccharide in the world, there is a lot more of the structural polysaccharide, cellulose. By some accounts half of all organic carbon is tied up in cellulose. An estimated 10
14
kilograms (about a 100 billion tons) of cellulose is biosynthesized and degraded annually. As it is not only an abundant but also a replenishable resource, the possibility of using cellulose as a cheap and readily available starting material for new products long interested chemists and entrepreneurs.
By the 1830s it was found that cellulose would dissolve in concentrated nitric acid and that this solution, when poured into water, formed a highly flammable and explosive white powder. Commercialization of this compound had to wait until 1845 and a discovery by Friedrich Schönbein of Basel, Switzerland. Schönbein was experimenting with mixtures of nitric and sulfuric acids in the kitchen of his home, against the wishes of his wife, who perhaps understandably had strictly forbidden the use of her residence for such activities. On this particular day his wife was out, and Schönbein spilled some of the acid mixture. Anxious to clean up the mess quickly, he grabbed the first thing that came to handâhis wife's cotton apron. He mopped up the spill and then hung the apron over the stove to dry. Before long, with an extremely loud bang and a great flash, the apron exploded. How Schönbein's wife reacted when she came home to find her husband continuing his kitchen experiments on cotton and the nitric acid mix is not known. What is recorded is what Schönbein called his materialâ
schiessbaumwolle,
or guncotton. Cotton is 90 percent cellulose, and we now know that Schönbein's guncotton was nitrocellulose, the compound formed when the nitro group (NO
2
) replaces the H of OH at a number of positions on the cellulose molecule. Not all these positions are necessarily nitrated, but the more nitration on cellulose, the more explosive is the guncotton produced.
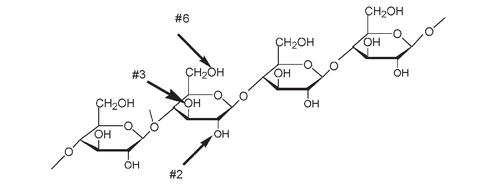
The structure of part of a cellulose molecule. The arrows show where nitration can take place at the OH on C#2, 3, and 6 of each of the glucose units
A portion of the structure of nitrocellulose or “guncotton” showing nitration;âNO
2
is substituted for -H at every possible OH position on each glucose unit of the cellulose.
Schönbein, recognizing the potential profit from his discovery, established factories to manufacture nitrocellulose, hoping it would become an alternative to gunpowder. But nitrocellulose can be an extremely dangerous compound unless it is kept dry and handled with proper care. At the time the destabilizing effect of residual nitric acid on the material was not understood, and thus a number of factories were accidentally destroyed by violent explosions, putting Schönbein out of business. It was not until the late 1860s, when proper methods were found to clean guncotton of excess nitric acid, that it could be made stable enough for use in commercial explosives.
Later, control of this nitration process led to different nitrocelluloses, including a higher-nitrate-content guncotton and the lower-nitrate-content materials collodion and celluloid. Collodion is a nitrocellulose mixed with alcohol and water and was used extensively in early photography. Celluloid, a nitrocellulose mixed with camphor, was one of the first successful plastics and was originally used as film for moving pictures. Another cellulose derivative, cellulose acetate, was found to be less flammable than nitrocellulose and quickly replaced it for many uses. The photography business and the movie industry, today enormous commercial enterprises, owe their beginnings to the chemical structure of the versatile cellulose molecule.
Cellulose is insoluble in almost all solvents but does dissolve in an alkaline solution of one organic solvent, carbon disulfide, forming a derivative of cellulose called cellulose xanthate. Cellulose xanthate is in the form of a viscous colloidal dispersion and was given the trade name of viscose. When viscose is forced through tiny holes and the resulting filament is treated with acid, the cellulose is regenerated in the form of fine threads that can be woven into a fabric known commercially as rayon. A similar process, where the viscose is extruded through a narrow slit, produces sheets of cellophane. Rayon and cellophane are usually considered to be synthetic textiles, but they are not totally man-made in the sense that they are just somewhat different forms derived from naturally occurring cellulose.